Probiotics and prebiotics in intestinal health and disease: from biology to the clinic
Probiotics and prebiotics are microbiota-management tools for improving host health. They target gastrointestinal effects via the gut, although direct application to other sites such as the oral cavity, vaginal tract and skin is being explored. Here, we describe gut-derived effects in humans. In the past decade, research on the gut microbiome has rapidly accumulated and has been accompanied by increased interest in probiotics and prebiotics as a means to modulate the gut microbiota. Given the importance of these approaches for public health, it is timely to reiterate factual and supporting information on their clinical application and use. In this Review, we discuss scientific evidence on probiotics and prebiotics, including mechanistic insights into health effects. Strains of Lactobacillus, Bifidobacterium and Saccharomyces have a long history of safe and effective use as probiotics, but Roseburia spp., Akkermansia spp., Propionibacterium spp. and Faecalibacterium spp. show promise for the future. For prebiotics, glucans and fructans are well proven, and evidence is building on the prebiotic effects of other substances (for example, oligomers of mannose, glucose, xylose, pectin, starches, human milk and polyphenols).
Key points
- The human gut microbiota is integral to health and is associated with a variety of diseases.
- Therapeutic and prophylactic effects of some probiotics and prebiotics for a variety of gut-related disorders might be, at least in part, mediated through modification of the microbiota and/or its function.
- Probiotic microorganisms act via a variety of means, including modulation of immune function, production of organic acids and antimicrobial compounds, interaction with resident microbiota, interfacing with the host, improving gut barrier integrity and enzyme formation.
- Prebiotics are substrates that are selectively utilized by host microorganisms conferring a health benefit; prebiotic effects include defence against pathogens, immune modulation, mineral absorption, bowel function, metabolic effects and satiety.
- Use of some probiotics and prebiotics is justified by robust assessments of efficacy, but not all products have been validated; the goal is evidence-based use by healthcare professionals.
This is a preview of subscription content, access via your institution
Access options
Access Nature and 54 other Nature Portfolio journals
Get Nature+, our best-value online-access subscription
cancel any time
Subscribe to this journal
Receive 12 print issues and online access
206,07 € per year
only 17,17 € per issue
Buy this article
- Purchase on SpringerLink
- Instant access to full article PDF
Prices may be subject to local taxes which are calculated during checkout
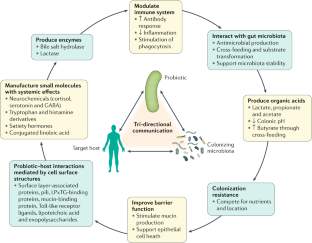
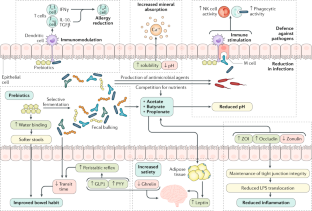
Similar content being viewed by others
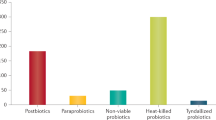
The International Scientific Association of Probiotics and Prebiotics (ISAPP) consensus statement on the definition and scope of postbiotics
Article Open access 04 May 2021
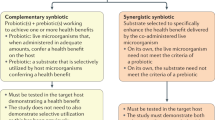
The International Scientific Association for Probiotics and Prebiotics (ISAPP) consensus statement on the definition and scope of synbiotics
Article Open access 21 August 2020
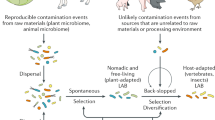
The International Scientific Association for Probiotics and Prebiotics (ISAPP) consensus statement on fermented foods
Article Open access 04 January 2021
Change history
References
- Food and Agriculture Organization of the United Nations & World Health Organization. Health and nutritional properties of probiotics in food including powder milk with live lactic acid bacteria. FAOhttp://www.fao.org/3/a-a0512e.pdf (2001).
- Hill, C. et al. Expert consensus document. The International Scientific Association for Probiotics and Prebiotics consensus statement on the scope and appropriate use of the term probiotic. Nat. Rev. Gastroenterol. Hepatol.11, 506–514 (2014). This Consensus Statement examines the definition, evolution, uses, types and health attributes of probiotics. PubMedGoogle Scholar
- Rook, G., Backhed, F., Levin, B. R., McFall-Ngai, M. J. & McLean, A. R. Evolution, human-microbe interactions, and life history plasticity. Lancet390, 521–530 (2017). This article discusses how some microorganisms have co-evolved with humans and have crucial roles in host physiology and metabolism, whereas others are intrusive. PubMedGoogle Scholar
- Reid, G. et al. Expanding the reach of probiotics through social enterprises. Benef. Microbes9, 707–715 (2018). CASPubMedGoogle Scholar
- Gibson, G. R. & Roberfroid, M. B. Dietary modulation of the human colonic microbiota: introducing the concept of prebiotics. J. Nutr.125, 1401–1412 (1995). CASPubMedGoogle Scholar
- Gibson, G. R. et al. Expert consensus document: The International Scientific Association for Probiotics and Prebiotics (ISAPP) consensus statement on the definition and scope of prebiotics. Nat. Rev. Gastroenterol. Hepatol.14, 491–502 (2017). This Consensus Statement examines the definition, evolution, uses, types and health attributes of prebiotics. PubMedGoogle Scholar
- Collins, S. L. et al. Promising prebiotic candidate established by evaluation of lactitol, lactulose, raffinose, and oligofructose for maintenance of a Lactobacillus-dominated vaginal microbiota. Appl. Environ. Microbiol.84, e02200-17 (2018). PubMedPubMed CentralGoogle Scholar
- Rodriguez, J. M. et al. The composition of the gut microbiota throughout life, with an emphasis on early life. Microb. Ecol. Health Dis.26, 26050 (2015). PubMedGoogle Scholar
- Donaldson, G. P., Lee, S. M. & Mazmanian, S. K. Gut biogeography of the bacterial microbiota. Nat. Rev. Microbiol.14, 20–32 (2016). CASPubMedGoogle Scholar
- Rowland, I. et al. Gut microbiota functions: metabolism of nutrients and other food components. Eur. J. Nutr.57, 1–24 (2018). CASPubMedGoogle Scholar
- Thursby, E. & Juge, N. Introduction to the human gut microbiota. Biochem. J.474, 1823–1836 (2017). This article provides current understanding of the development and composition of the human gut microbiota, and its effects on gut integrity and host health. CASPubMedGoogle Scholar
- Fava, F. et al. The type and quantity of dietary fat and carbohydrate alter faecal microbiome and short-chain fatty acid excretion in a metabolic syndrome ‘at-risk’ population. Int. J. Obes. (Lond.)37, 216–223 (2013). CASGoogle Scholar
- Dicks, L. M. T., Geldenhuys, J., Mikkelsen, L. S., Brandsborg, E. & Marcotte, H. Our gut microbiota: a long walk to homeostasis. Benef. Microbes9, 3–20 (2018). CASPubMedGoogle Scholar
- Dethlefsen, L. & Relman, D. A. Incomplete recovery and individualized responses of the human distal gut microbiota to repeated antibiotic perturbation. Proc. Natl Acad. Sci. U. S. A.108 (Suppl. 1), 4554–4561 (2011). CASPubMedGoogle Scholar
- Gagliardi, A. et al. Rebuilding the gut microbiota ecosystem. Int. J. Environ. Res. Public Health15, E1679 (2018). PubMedGoogle Scholar
- Hatton, G. B., Madla, C. M., Rabbie, S. C. & Basit, A. W. All disease begins in the gut: influence of gastrointestinal disorders and surgery on oral drug performance. Int. J. Pharm.548, 408–422 (2018). CASPubMedGoogle Scholar
- John, G. K. et al. Dietary alteration of the gut microbiome and its impact on weight and fat mass: a systematic review and meta-analysis. Genes (Basel)9, (E167 (2018). Google Scholar
- Yoshida, N., Yamashita, T. & Hirata, K. I. Gut microbiome and cardiovascular diseases. Diseases6, E56 (2018). PubMedGoogle Scholar
- Hansen, L. B. S. et al. A low-gluten diet induces changes in the intestinal microbiome of healthy Danish adults. Nat. Commun.9, 4630 (2018). PubMedPubMed CentralGoogle Scholar
- Parker, R. B. Probiotics, the other half of the antibiotic story. Anim. Nutr. Health29, 4–8 (1974). Google Scholar
- Havenaar, R. & Huis In’t Veld, J. M. J. in Lactic Acid Bacteria in Health and Disease Vol. 1 (ed. Wood, B. J. B.) 151–170 (Elsevier Applied Science Publishers, 1992).
- Ng, S. C. et al. Immunosuppressive effects via human intestinal dendritic cells of probiotic bacteria and steroids in the treatment of acute ulcerative colitis. Inflamm. Bowel Dis.16, 1286–1298 (2010). PubMedGoogle Scholar
- Mujagic, Z. et al. The effects of Lactobacillus plantarum on small intestinal barrier function and mucosal gene transcription; a randomized double-blind placebo controlled trial. Sci. Rep.7, 40128 (2017). CASPubMedPubMed CentralGoogle Scholar
- Del Piano, M. et al. The use of probiotics in healthy volunteers with evacuation disorders and hard stools: a double-blind, randomized, placebo-controlled study. J. Clin. Gastroenterol.44 (Suppl. 1), S30–S34 (2010). PubMedGoogle Scholar
- Reid, G., Gadir, A. A. & Dhir, R. Probiotics: reiterating what they are and what they are not. Front. Microbiol.10, 424 (2019). PubMedPubMed CentralGoogle Scholar
- Cabre, E. & Gassull, M. A. Probiotics for preventing relapse or recurrence in Crohn’s disease involving the ileum: are there reasons for failure? J. Crohns Colitis1, 47–52 (2007). PubMedGoogle Scholar
- Kelly, J. R. et al. Lost in translation? The potential psychobiotic Lactobacillus rhamnosus (JB-1) fails to modulate stress or cognitive performance in healthy male subjects. Brain Behav. Immun.61, 50–59 (2017). CASPubMedGoogle Scholar
- Panigrahi, P. et al. A randomized synbiotic trial to prevent sepsis among infants in rural India. Nature548, 407–412 (2017). CASPubMedGoogle Scholar
- Costeloe, K. et al. Bifidobacterium breve BBG-001 in very preterm infants: a randomised controlled phase 3 trial. Lancet387, 649–660 (2016). PubMedGoogle Scholar
- Sorbara, M. T. & Pamer, E. G. Interbacterial mechanisms of colonization resistance and the strategies pathogens use to overcome them. Mucosal Immunol.34, 1608 (2018). Google Scholar
- Chiu, L. et al. Protective microbiota: from localized to long-reaching co-immunity. Front. Immunol.8, 1678 (2017). PubMedPubMed CentralGoogle Scholar
- Maldonado-Gomez, M. X. et al. Stable engraftment of Bifidobacterium longum AH1206 in the human gut depends on individualized features of the resident microbiome. Cell Host Microbe20, 515–526 (2016). CASPubMedGoogle Scholar
- Murphy, R. et al. Eczema-protective probiotic alters infant gut microbiome functional capacity but not composition: sub-sample analysis from a RCT. Benef. Microbes10, 5–17 (2019). CASPubMedGoogle Scholar
- Korpela, K. et al. Probiotic supplementation restores normal microbiota composition and function in antibiotic-treated and in caesarean-born infants. Microbiome6, 182 (2018). PubMedPubMed CentralGoogle Scholar
- Clarke, G. et al. Gut reactions: breaking down xenobiotic-microbiome interactions. Pharmacol. Rev.71, 198–224 (2019). PubMedGoogle Scholar
- Klaenhammer, T. R., Kleerebezem, M., Kopp, M. V. & Rescigno, M. The impact of probiotics and prebiotics on the immune system. Nat. Rev. Immunol.12, 728–734 (2012). Here, four experts discuss probiotics, prebiotics and immunity, then provide their thoughts on the future application as a disease therapy. CASPubMedGoogle Scholar
- Przemska-Kosicka, A. et al. Effect of a synbiotic on the response to seasonal influenza vaccination is strongly influenced by degree of immunosenescence. Immun. Ageing13, 6 (2016). PubMedPubMed CentralGoogle Scholar
- Vitetta, L., Saltzman, E. T., Thomsen, M., Nikov, T. & Hall, S. Adjuvant probiotics and the intestinal microbiome: enhancing vaccines and immunotherapy outcomes. Vaccines (Basel) 5, (E50 (2017). Google Scholar
- Childs, C. E. et al. Xylo-oligosaccharides alone or in synbiotic combination with Bifidobacterium animalis subsp. lactis induce bifidogenesis and modulate markers of immune function in healthy adults: a double-blind, placebo-controlled, randomised, factorial cross-over study. Br. J. Nutr.111, 1945–1956 (2014). CASPubMedGoogle Scholar
- Flint, H. J., Duncan, S. H., Scott, K. P. & Louis, P. Links between diet, gut microbiota composition and gut metabolism. Proc. Nutr. Soc.74, 13–22 (2015). CASPubMedGoogle Scholar
- Aoudia, N. et al. Biofilms of Lactobacillus plantarum and Lactobacillus fermentum: effect on stress responses, antagonistic effects on pathogen growth and immunomodulatory properties. Food Microbiol.53, 51–59 (2016). CASPubMedGoogle Scholar
- Rios-Covian, D. et al. Intestinal short chain fatty acids and their link with diet and human health. Front. Microbiol.7, 185 (2016). PubMedPubMed CentralGoogle Scholar
- Canfora, E. E., Jocken, J. W. & Blaak, E. E. Short-chain fatty acids in control of body weight and insulin sensitivity. Nat. Rev. Endocrinol.11, 577–591 (2015). CASPubMedGoogle Scholar
- Sanna, S. et al. Causal relationships among the gut microbiome, short-chain fatty acids and metabolic diseases. Nat. Genet.51, 600–605 (2019). CASPubMedPubMed CentralGoogle Scholar
- Stefan, N., Fritsche, A., Schick, F. & Haring, H. U. Phenotypes of prediabetes and stratification of cardiometabolic risk. Lancet Diabetes Endocrinol.4, 789–798 (2016). PubMedGoogle Scholar
- van Baarlen, P., Wells, J. M. & Kleerebezem, M. Regulation of intestinal homeostasis and immunity with probiotic lactobacilli. Trends Immunol.34, 208–215 (2013). PubMedGoogle Scholar
- Hegarty, J. W., Guinane, C. M., Ross, R. P., Hill, C. & Cotter, P. D. Bacteriocin production: a relatively unharnessed probiotic trait? F1000Res.5, 2587 (2016). PubMedPubMed CentralGoogle Scholar
- Mokoena, M. P. Lactic acid bacteria and their bacteriocins: classification, biosynthesis and applications against uropathogens: a mini-review. Molecules22, E1255 (2017). PubMedGoogle Scholar
- Bali, V., Panesar, P. S., Bera, M. B. & Kennedy, J. F. Bacteriocins: recent trends and potential applications. Crit. Rev. Food Sci. Nutr.56, 817–834 (2016). CASPubMedGoogle Scholar
- Riviere, A., Selak, M., Lantin, D., Leroy, F. & De Vuyst, L. Bifidobacteria and butyrate-producing colon bacteria: importance and strategies for their stimulation in the human gut. Front. Microbiol.7, 979 (2016). PubMedPubMed CentralGoogle Scholar
- Abdulkadir, B. et al. Routine use of probiotics in preterm infants: longitudinal impact on the microbiome and metabolome. Neonatology109, (239–247 (2016). Google Scholar
- Fang, H. R., Zhang, G. Q., Cheng, J. Y. & Li, Z. Y. Efficacy of Lactobacillus-supplemented triple therapy for Helicobacter pylori infection in children: a meta-analysis of randomized controlled trials. Eur. J. Pediatr.178, 7–16 (2019). CASPubMedGoogle Scholar
- Sanders, M. E., Benson, A., Lebeer, S., Merenstein, D. J. & Klaenhammer, T. R. Shared mechanisms among probiotic taxa: implications for general probiotic claims. Curr. Opin. Biotechnol.49, 207–216 (2018). CASPubMedGoogle Scholar
- Petrova, M. I. et al. Comparative genomic and phenotypic analysis of the vaginal probiotic Lactobacillus rhamnosus GR-1. Front. Microbiol.9, 1278 (2018). PubMedPubMed CentralGoogle Scholar
- La Fata, G., Weber, P. & Mohajeri, M. H. Probiotics and the gut immune system: indirect regulation. Probiot. Antimicrob. Proteins10, 11–21 (2018). Google Scholar
- Han, X. et al. Lactobacillus rhamnosus GG prevents epithelial barrier dysfunction induced by interferon-gamma and fecal supernatants from irritable bowel syndrome patients in human intestinal enteroids and colonoids. Gut Microbes10, 59–76 (2019). CASPubMedGoogle Scholar
- Mack, D. R., Michail, S., Wei, S., McDougall, L. & Hollingsworth, M. A. Probiotics inhibit enteropathogenic E. coli adherence in vitro by inducing intestinal mucin gene expression. Am. J. Physiol.276, G941–G950 (1999). CASPubMedGoogle Scholar
- Yan, F. et al. A Lactobacillus rhamnosus GG-derived soluble protein, p40, stimulates ligand release from intestinal epithelial cells to transactivate epidermal growth factor receptor. J. Biol. Chem.288, 30742–30751 (2013). CASPubMedPubMed CentralGoogle Scholar
- Stadlbauer, V. et al. Lactobacillus casei Shirota supplementation does not restore gut microbiota composition and gut barrier in metabolic syndrome: a randomized pilot study. PLOS ONE10, e0141399 (2015). PubMedPubMed CentralGoogle Scholar
- Kim, N., Yun, M., Oh, Y. J. & Choi, H. J. Mind-altering with the gut: modulation of the gut-brain axis with probiotics. J. Microbiol.56, 172–182 (2018). CASPubMedGoogle Scholar
- Janik, R. et al. Magnetic resonance spectroscopy reveals oral Lactobacillus promotion of increases in brain GABA, N-acetyl aspartate and glutamate. Neuroimage125, 988–995 (2016). CASPubMedGoogle Scholar
- Reid, G. Disentangling what we know about microbes and mental health. Front. Endocrinol.10, 81 (2019). Google Scholar
- Liang, S. et al. Administration of Lactobacillus helveticus NS8 improves behavioral, cognitive, and biochemical aberrations caused by chronic restraint stress. Neuroscience310, 561–577 (2015). CASPubMedGoogle Scholar
- Kotz, C. M., Furne, J. K., Savaiano, D. A. & Levitt, M. D. Factors affecting the ability of a high beta-galactosidase yogurt to enhance lactose absorption. J. Dairy Sci.77, 3538–3544 (1994). CASPubMedGoogle Scholar
- Costabile, A. et al. An in vivo assessment of the cholesterol-lowering efficacy of Lactobacillus plantarum ECGC 13110402 in normal to mildly hypercholesterolaemic adults. PLOS ONE12, e0187964 (2017). PubMedPubMed CentralGoogle Scholar
- European Food Safety Authority Panel on Dietetic Products. Scientific opinion on the substantiation of health claims related to live yoghurt cultures and improved lactose digestion (ID 1143, 2976) pursuant to article 13(1) of regulation (EC) No 1924/2006. EFSA J.8, 1763 (2010). Google Scholar
- Li, D., Wang, P., Wang, P., Hu, X. & Chen, F. The gut microbiota: a treasure for human health. Biotechnol. Adv.34, 1210–1224 (2016). PubMedGoogle Scholar
- Kasubuchi, M., Hasegawa, S., Hiramatsu, T., Ichimura, A. & Kimura, I. Dietary gut microbial metabolites, short-chain fatty acids, and host metabolic regulation. Nutrients7, 2839–2849 (2015). CASPubMedPubMed CentralGoogle Scholar
- Verbeke, K. A. et al. Towards microbial fermentation metabolites as markers for health benefits of prebiotics. Nutr. Res. Rev.28, 42–66 (2015). CASPubMedPubMed CentralGoogle Scholar
- David, L. A. et al. Diet rapidly and reproducibly alters the human gut microbiome. Nature505, 559–563 (2014). CASPubMedGoogle Scholar
- Fogliano, V. et al. In vitro bioaccessibility and gut biotransformation of polyphenols present in the water-insoluble cocoa fraction. Mol. Nutr. Food Res.55 (Suppl. 1), S44–S55 (2011). CASPubMedGoogle Scholar
- Falony, G. et al. In vitro kinetic analysis of fermentation of prebiotic inulin-type fructans by Bifidobacterium species reveals four different phenotypes. Appl. Environ. Microbiol.75, 454–461 (2009). CASPubMedGoogle Scholar
- Riviere, A., Selak, M., Geirnaert, A., Van den Abbeele, P. & De Vuyst, L. Complementary mechanisms for degradation of inulin-type fructans and arabinoxylan oligosaccharides among bifidobacterial strains suggest bacterial cooperation. Appl. Environ. Microbiol.84, e02893-17 (2018). CASPubMedPubMed CentralGoogle Scholar
- Flint, H. J., Scott, K. P., Duncan, S. H., Louis, P. & Forano, E. Microbial degradation of complex carbohydrates in the gut. Gut Microbes3, 289–306 (2012). PubMedPubMed CentralGoogle Scholar
- Hamaker, B. R. & Tuncil, Y. E. A perspective on the complexity of dietary fiber structures and their potential effect on the gut microbiota. J. Mol. Biol.426, 3838–3850 (2014). CASPubMedGoogle Scholar
- Ze, X., Le Mougen, F., Duncan, S. H., Louis, P. & Flint, H. J. Some are more equal than others: the role of “keystone” species in the degradation of recalcitrant substrates. Gut Microbes4, 236–240 (2013). PubMedPubMed CentralGoogle Scholar
- Ze, X., Duncan, S. H., Louis, P. & Flint, H. J. Ruminococcus bromii is a keystone species for the degradation of resistant starch in the human colon. ISME J.6, 1535–1543 (2012). CASPubMedPubMed CentralGoogle Scholar
- Hosseini, E., Grootaert, C., Verstraete, W. & Van de Wiele, T. Propionate as a health-promoting microbial metabolite in the human gut. Nutr. Rev.69, 245–258 (2011). PubMedGoogle Scholar
- Louis, P. & Flint, H. J. Diversity, metabolism and microbial ecology of butyrate-producing bacteria from the human large intestine. FEMS Microbiol. Lett.294, 1–8 (2009). CASPubMedGoogle Scholar
- Falony, G., Calmeyn, T., Leroy, F. & De Vuyst, L. Coculture fermentations of Bifidobacterium species and Bacteroides thetaiotaomicron reveal a mechanistic insight into the prebiotic effect of inulin-type fructans. Appl. Environ. Microbiol.75, 2312–2319 (2009). CASPubMedPubMed CentralGoogle Scholar
- Scott, K. P., Martin, J. C., Duncan, S. H. & Flint, H. J. Prebiotic stimulation of human colonic butyrate-producing bacteria and bifidobacteria, in vitro. FEMS Microbiol. Ecol.87, 30–40 (2014). CASPubMedGoogle Scholar
- Flint, H. J., Duncan, S. H. & Louis, P. The impact of nutrition on intestinal bacterial communities. Curr. Opin. Microbiol.38, 59–65 (2017). CASPubMedGoogle Scholar
- Chen, T. et al. Fiber-utilizing capacity varies in Prevotella- versus Bacteroides-dominated gut microbiota. Sci. Rep.7, 2594 (2017). PubMedPubMed CentralGoogle Scholar
- Wu, Q. et al. Fermentation properties of isomaltooligosaccharides are affected by human fecal enterotypes. Anaerobe48, 206–214 (2017). CASPubMedGoogle Scholar
- Qin, J. et al. A human gut microbial gene catalogue established by metagenomic sequencing. Nature464, 59–65 (2010). CASPubMedPubMed CentralGoogle Scholar
- Frank, D. N. et al. Molecular-phylogenetic characterization of microbial community imbalances in human inflammatory bowel diseases. Proc. Natl Acad. Sci. U. S. A.104, 13780–13785 (2007). CASPubMedPubMed CentralGoogle Scholar
- Larsen, N. et al. Gut microbiota in human adults with type 2 diabetes differs from non-diabetic adults. PLOS ONE5, e9085 (2010). PubMedPubMed CentralGoogle Scholar
- Qin, J. et al. A metagenome-wide association study of gut microbiota in type 2 diabetes. Nature490, 55–60 (2012). This study provides a definition and description of the minimal gut metagenome and bacterial genome in terms of functions. CASPubMedGoogle Scholar
- Karlsson, F. H. et al. Gut metagenome in European women with normal, impaired and diabetic glucose control. Nature498, 99–103 (2013). CASPubMedGoogle Scholar
- Carroll, I. M., Chang, Y. H., Park, J., Sartor, R. B. & Ringel, Y. Luminal and mucosal-associated intestinal microbiota in patients with diarrhea-predominant irritable bowel syndrome. Gut Pathog.2, 19 (2010). PubMedPubMed CentralGoogle Scholar
- Krogius-Kurikka, L. et al. Microbial community analysis reveals high level phylogenetic alterations in the overall gastrointestinal microbiota of diarrhoea-predominant irritable bowel syndrome sufferers. BMC Gastroenterol.9, 95 (2009). PubMedPubMed CentralGoogle Scholar
- Turnbaugh, P. J. et al. An obesity-associated gut microbiome with increased capacity for energy harvest. Nature444, 1027–1031 (2006). PubMedGoogle Scholar
- Zhang, H. et al. Human gut microbiota in obesity and after gastric bypass. Proc. Natl Acad. Sci. U. S. A.106, 2365–2370 (2009). CASPubMedPubMed CentralGoogle Scholar
- Ha, C. W., Lam, Y. Y. & Holmes, A. J. Mechanistic links between gut microbial community dynamics, microbial functions and metabolic health. World J. Gastroenterol.20, 16498–16517 (2014). CASPubMedPubMed CentralGoogle Scholar
- Moya, A. & Ferrer, M. Functional redundancy-induced stability of gut microbiota subjected to disturbance. Trends Microbiol.24, 402–413 (2016). CASPubMedGoogle Scholar
- Fooks, L. J. & Gibson, G. R. In vitro investigations of the effect of probiotics and prebiotics on selected human intestinal pathogens. FEMS Microbiol. Ecol.39, 67–75 (2002). CASPubMedGoogle Scholar
- Tzortzis, G., Baillon, M. L., Gibson, G. R. & Rastall, R. A. Modulation of anti-pathogenic activity in canine-derived Lactobacillus species by carbohydrate growth substrate. J. Appl. Microbiol.96, 552–559 (2004). CASPubMedGoogle Scholar
- Vulevic, J., Drakoularakou, A., Yaqoob, P., Tzortzis, G. & Gibson, G. R. Modulation of the fecal microflora profile and immune function by a novel trans-galactooligosaccharide mixture (B-GOS) in healthy elderly volunteers. Am. J. Clin. Nutr.88, 1438–1446 (2008). CASPubMedGoogle Scholar
- Vulevic, J. et al. Influence of galacto-oligosaccharide mixture (B-GOS) on gut microbiota, immune parameters and metabonomics in elderly persons. Br. J. Nutr.114, 586–595 (2015). CASPubMedGoogle Scholar
- Moro, G. et al. A mixture of prebiotic oligosaccharides reduces the incidence of atopic dermatitis during the first six months of age. Arch. Dis. Child.91, 814–819 (2006). CASPubMedPubMed CentralGoogle Scholar
- Ivakhnenko, O. S. & Nyankovskyy, S. L. Effect of the specific infant formula mixture of oligosaccharides on local immunity and development of allergic and infectious disease in young children: randomized study. Pediatr. Pol.88, 398–404 (2013). Google Scholar
- Arslanoglu, S. et al. Early neutral prebiotic oligosaccharide supplementation reduces the incidence of some allergic manifestations in the first 5 years of life. J. Biol. Regul. Homeost. Agents26, 49–59 (2012). CASPubMedGoogle Scholar
- Diaz de Barboza, G. & Guizzardi, S. & Tolosa de Talamoni, N. Molecular aspects of intestinal calcium absorption. World J. Gastroenterol.21, 7142–7154 (2015). CASPubMedPubMed CentralGoogle Scholar
- Goss, S. L., Lemons, K. A., Kerstetter, J. E. & Bogner, R. H. Determination of calcium salt solubility with changes in pH and P(CO(2)), simulating varying gastrointestinal environments. J. Pharm. Pharmacol.59, 1485–1492 (2007). PubMedGoogle Scholar
- Abrams, S. A., Griffin, I. J., Hawthorne, K. M. & Ellis, K. J. Effect of prebiotic supplementation and calcium intake on body mass index. J. Pediatr.151, 293–298 (2007). CASPubMedGoogle Scholar
- Abrams, S. A., Griffin, I. J. & Hawthorne, K. M. Young adolescents who respond to an inulin-type fructan substantially increase total absorbed calcium and daily calcium accretion to the skeleton. J. Nutr.137, 2524S–2526S (2007). CASPubMedGoogle Scholar
- Whisner, C. M. et al. Galacto-oligosaccharides increase calcium absorption and gut bifidobacteria in young girls: a double-blind cross-over trial. Br. J. Nutr.110, 1292–1303 (2013). CASPubMedGoogle Scholar
- Chonan, O., Matsumoto, K. & Watanuki, M. Effect of galactooligosaccharides on calcium absorption and preventing bone loss in ovariectomized rats. Biosci. Biotechnol. Biochem.59, 236–239 (1995). CASPubMedGoogle Scholar
- Kanauchi, O., Andoh, A. & Mitsuyama, K. Effects of the modulation of microbiota on the gastrointestinal immune system and bowel function. J. Agric. Food Chem.61, 9977–9983 (2013). CASPubMedGoogle Scholar
- Hurst, N. R., Kendig, D. M., Murthy, K. S. & Grider, J. R. The short chain fatty acids, butyrate and propionate, have differential effects on the motility of the guinea pig colon. Neurogastroenterol. Motil.26, 1586–1596 (2014). CASPubMedPubMed CentralGoogle Scholar
- Lamsal, B. P. Production, health aspects and potential food uses of dairy prebiotic galactooligosaccharides. J. Sci. Food Agric.92, 2020–2028 (2012). CASPubMedGoogle Scholar
- Hager, A.-S. et al. Influence of the soluble fibres inulin and oat β-glucan on quality of dough and bread. Eur. Food Res. Technol.232, 405–413 (2011). CASGoogle Scholar
- Collado Yurrita, L., San Mauro Martin, I., Ciudad-Cabanas, M. J., Calle-Puron, M. E. & Hernandez Cabria, M. Effectiveness of inulin intake on indicators of chronic constipation; a meta-analysis of controlled randomized clinical trials. Nutr. Hosp.30, 244–252 (2014). PubMedGoogle Scholar
- Buddington, R. K., Kapadia, C., Neumer, F. & Theis, S. Oligofructose provides laxation for irregularity associated with low fiber intake. Nutrients9, E1372 (2017). PubMedGoogle Scholar
- Krumbeck, J. A. et al. Probiotic Bifidobacterium strains and galactooligosaccharides improve intestinal barrier function in obese adults but show no synergism when used together as synbiotics. Microbiome6, 121 (2018). PubMedPubMed CentralGoogle Scholar
- Cani, P. D. et al. Metabolic endotoxemia initiates obesity and insulin resistance. Diabetes56, 1761–1772 (2007). CASPubMedGoogle Scholar
- Cani, P. D. et al. Changes in gut microbiota control metabolic endotoxemia-induced inflammation in high-fat diet-induced obesity and diabetes in mice. Diabetes57, 1470–1481 (2008). CASPubMedGoogle Scholar
- Cani, P. D. et al. Changes in gut microbiota control inflammation in obese mice through a mechanism involving GLP-2-driven improvement of gut permeability. Gut58, 1091–1103 (2009). CASPubMedGoogle Scholar
- Kellow, N. J., Coughlan, M. T. & Reid, C. M. Metabolic benefits of dietary prebiotics in human subjects: a systematic review of randomised controlled trials. Br. J. Nutr.111, 1147–1161 (2014). CASPubMedGoogle Scholar
- Beserra, B. T. et al. A systematic review and meta-analysis of the prebiotics and synbiotics effects on glycaemia, insulin concentrations and lipid parameters in adult patients with overweight or obesity. Clin. Nutr.34, 845–858 (2015). CASPubMedGoogle Scholar
- Liu, F., Prabhakar, M., Ju, J., Long, H. & Zhou, H. W. Effect of inulin-type fructans on blood lipid profile and glucose level: a systematic review and meta-analysis of randomized controlled trials. Eur. J. Clin. Nutr.71, 9–20 (2017). CASPubMedGoogle Scholar
- Guo, Z. et al. Effects of inulin on the plasma lipid profile of normolipidemic and hyperlipidemic subjects: a meta-analysis of randomized controlled trials. Clin. Lipidol7, 215–222 (2012). CASGoogle Scholar
- Vulevic, J., Juric, A., Tzortzis, G. & Gibson, G. R. A mixture of trans-galactooligosaccharides reduces markers of metabolic syndrome and modulates the fecal microbiota and immune function of overweight adults. J. Nutr.143, 324–331 (2013). CASPubMedGoogle Scholar
- Dewulf, E. M. et al. Insight into the prebiotic concept: lessons from an exploratory, double blind intervention study with inulin-type fructans in obese women. Gut62, 1112–1121 (2013). CASPubMedGoogle Scholar
- Bhatia, S. et al. Galacto-oligosaccharides may directly enhance intestinal barrier function through the modulation of goblet cells. Mol. Nutr. Food Res.59, 566–573 (2015). CASPubMedGoogle Scholar
- Akbari, P. et al. Characterizing microbiota-independent effects of oligosaccharides on intestinal epithelial cells: insight into the role of structure and size: structure-activity relationships of non-digestible oligosaccharides. Eur. J. Nutr.56, 1919–1930 (2017). CASPubMedGoogle Scholar
- Neyrinck, A. M. et al. Intestinal sucrase as a novel target contributing to the regulation of glycemia by prebiotics. PLOS ONE11, e0160488 (2016). PubMedPubMed CentralGoogle Scholar
- Stoddart, L. A., Smith, N. J. & Milligan, G. International Union of Pharmacology. LXXI. Free fatty acid receptors FFA1, -2, and -3: pharmacology and pathophysiological functions. Pharmacol. Rev.60, 405–417 (2008). CASPubMedGoogle Scholar
- Bolognini, D. et al. Chemogenetics defines receptor-mediated functions of short chain free fatty acids. Nat. Chem. Biol.15, 489–498 (2019). CASPubMedGoogle Scholar
- Chambers, E. S., Morrison, D. J. & Frost, G. Control of appetite and energy intake by SCFA: what are the potential underlying mechanisms? Proc. Nutr. Soc.74, 328–336 (2015). CASPubMedGoogle Scholar
- Mithieux, G. Metabolic effects of portal vein sensing. Diabetes Obes. Metab.16 (Suppl. 1), 56–60 (2014). CASPubMedGoogle Scholar
- Frost, G. et al. The short-chain fatty acid acetate reduces appetite via a central homeostatic mechanism. Nat. Commun.5, 3611 (2014). CASPubMedGoogle Scholar
- Jackson, S. A. et al. Improving end-user trust in the quality of commercial probiotic products. Front. Microbiol.10, 739 (2019). PubMedPubMed CentralGoogle Scholar
- AlFaleh, K. & Anabrees, J. Probiotics for prevention of necrotizing enterocolitis in preterm infants. Cochrane Database Syst. Rev.4, CD005496 (2014). Google Scholar
- Vanderhoof, J. A. et al. Lactobacillus GG in the prevention of antibiotic-associated diarrhea in children. J. Pediatr.135, 564–568 (1999). CASPubMedGoogle Scholar
- Szajewska, H., Albrecht, P. & Topczewska-Cabanek, A. Randomized, double-blind, placebo-controlled trial: effect of Lactobacillus GG supplementation on Helicobacter pylori eradication rates and side effects during treatment in children. J. Pediatr. Gastroenterol. Nutr.48, 431–436 (2009). CASPubMedGoogle Scholar
- Goldenberg, J. Z. et al. Probiotics for the prevention of pediatric antibiotic-associated diarrhea. Cochrane Database Syst. Rev.4, CD004827 (2015). Google Scholar
- Eskesen, D. et al. Effect of the probiotic strain Bifidobacterium animalis subsp. lactis, BB-12®, on defecation frequency in healthy subjects with low defecation frequency and abdominal discomfort: a randomised, double-blind, placebo-controlled, parallel-group trial. Br. J. Nutr.114, 1638–1646 (2015). CASPubMedPubMed CentralGoogle Scholar
- Yang, Y. X. et al. Effect of a fermented milk containing Bifidobacterium lactis DN-173010 on Chinese constipated women. World J. Gastroenterol.14, 6237–6243 (2008). PubMedPubMed CentralGoogle Scholar
- Sung, V. et al. Lactobacillus reuteri to treat infant colic: a meta-analysis. Pediatrics141, e20171811 (2018). PubMedGoogle Scholar
- Mardini, H. E. & Grigorian, A. Y. Probiotic mix VSL#3 is effective adjunctive therapy for mild to moderately active ulcerative colitis: a meta-analysis. Inflamm. Bowel Dis.20, 1562–1567 (2014). PubMedGoogle Scholar
- Whorwell, P. J. et al. Efficacy of an encapsulated probiotic Bifidobacterium infantis 35624 in women with irritable bowel syndrome. Am. J. Gastroenterol.101, 1581–1590 (2006). PubMedGoogle Scholar
- Szajewska, H. et al. Systematic review with meta-analysis: Lactobacillus rhamnosus GG for treating acute gastroenteritis in children - a 2019 update. Aliment. Pharmacol. Ther.49, 1376–1384 (2019). PubMedGoogle Scholar
- Goldenberg, J. Z. et al. Probiotics for the prevention of Clostridium difficile-associated diarrhea in adults and children. Cochrane Database Syst. Rev.12, CD006095 (2017). PubMedGoogle Scholar
- King, S. et al. Does probiotic consumption reduce antibiotic utilization for common acute infections? A systematic review and meta-analysis. Eur. J. Public Health29, 494–499 (2019). PubMedGoogle Scholar
- Cruchet, S. et al. The use of probiotics in pediatric gastroenterology: a review of the literature and recommendations by Latin-American experts. Paediatr. Drugs17, 199–216 (2015). PubMedPubMed CentralGoogle Scholar
- Cameron, D. et al. Probiotics for gastrointestinal disorders: proposed recommendations for children of the Asia-Pacific region. World J. Gastroenterol.23, 7952–7964 (2017). PubMedPubMed CentralGoogle Scholar
- Hao, Q., Dong, B. R. & Wu, T. Probiotics for preventing acute upper respiratory tract infections. Cochrane Database Syst. Rev.9, CD006895 (2015). Google Scholar
- King, S., Glanville, J., Sanders, M. E., Fitzgerald, A. & Varley, D. Effectiveness of probiotics on the duration of illness in healthy children and adults who develop common acute respiratory infectious conditions: a systematic review and meta-analysis. Br. J. Nutr.112, 41–54 (2014). CASPubMedPubMed CentralGoogle Scholar
- Yang, L. et al. Inflammation and intestinal metaplasia of the distal esophagus are associated with alterations in the microbiome. Gastroenterology137, 588–597 (2009). PubMedGoogle Scholar
- Szymanski, H. & Szajewska, H. Lack of efficacy of Lactobacillus reuteri DSM 17938 for the treatment of acute gastroenteritis: a randomized controlled trial. Pediatr. Infect. Dis. J.https://doi.org/10.1097/INF.0000000000002355 (2019). ArticlePubMedGoogle Scholar
- van den Akker, C. H. P. et al. Probiotics for preterm infants: a strain-specific systematic review and network meta-analysis. J. Pediatr. Gastroenterol. Nutr.67, 103–122 (2018). PubMedGoogle Scholar
- Arslanoglu, S., Moro, G. E. & Boehm, G. Early supplementation of prebiotic oligosaccharides protects formula-fed infants against infections during the first 6 months of life. J. Nutr.137, 2420–2424 (2007). CASPubMedGoogle Scholar
- Arslanoglu, S. et al. Early dietary intervention with a mixture of prebiotic oligosaccharides reduces the incidence of allergic manifestations and infections during the first two years of life. J. Nutr.138, 1091–1095 (2008). CASPubMedGoogle Scholar
- Boehm, G. et al. Prebiotics in infant formulas. J. Clin. Gastroenterol.38, S76–S79 (2004). CASPubMedGoogle Scholar
- Shahramian, I. et al. The effects of prebiotic supplementation on weight gain, diarrhoea, constipation, fever and respiratory tract infections in the first year of life. J. Paediatr. Child Health54, 875–880 (2018). PubMedGoogle Scholar
- Drakoularakou, A., Tzortzis, G., Rastall, R. A. & Gibson, G. R. A double-blind, placebo-controlled, randomized human study assessing the capacity of a novel galacto-oligosaccharide mixture in reducing travellers’ diarrhoea. Eur. J. Clin. Nutr.64, 146–152 (2010). CASPubMedGoogle Scholar
- Micka, A., Siepelmeyer, A., Holz, A., Theis, S. & Schon, C. Effect of consumption of chicory inulin on bowel function in healthy subjects with constipation: a randomized, double-blind, placebo-controlled trial. Int. J. Food Sci. Nutr.68, 82–89 (2017). CASPubMedGoogle Scholar
- European Food Safety Authority Panel on Dietetic Products. Scientific opinion on the substantiation of a health claim related to “native chicory inulin” and maintenance of normal defecation by increasing stool frequency pursuant to article 13.5 of regulation (EC) No 1924/2006. EFSA J.13, 3951 (2015). Google Scholar
- Hume, M. P., Nicolucci, A. C. & Reimer, R. A. Prebiotic supplementation improves appetite control in children with overweight and obesity: a randomized controlled trial. Am. J. Clin. Nutr.105, 790–799 (2017). CASPubMedGoogle Scholar
- Nicolucci, A. C. et al. Prebiotics reduce body fat and alter intestinal microbiota in children who are overweight or with obesity. Gastroenterology153, 711–722 (2017). PubMedGoogle Scholar
- Pol, K., de Graaf, C., Meyer, D. & Mars, M. The efficacy of daily snack replacement with oligofructose-enriched granola bars in overweight and obese adults: a 12-week randomised controlled trial. Br. J. Nutr.119, 1076–1086 (2018). CASPubMedGoogle Scholar
- Liber, A. & Szajewska, H. Effect of oligofructose supplementation on body weight in overweight and obese children: a randomised, double-blind, placebo-controlled trial. Br. J. Nutr.112, 2068–2074 (2014). CASPubMedGoogle Scholar
- European Food Safety Authority Panel on Dietetic Products. Scientific opinion on the substantiation of a health claim related to non-digestible carbohydrates and a reduction of post-prandial glycaemic responses pursuant to article 13(5) of regulation (EC) No 1924/2006. EFSA J.12, 3513 (2015). Google Scholar
- Lightowler, H., Thondre, S., Holz, A. & Theis, S. Replacement of glycaemic carbohydrates by inulin-type fructans from chicory (oligofructose, inulin) reduces the postprandial blood glucose and insulin response to foods: report of two double-blind, randomized, controlled trials. Eur. J. Nutr.57, 1259–1268 (2018). CASPubMedGoogle Scholar
- Olbjorn, C. et al. Fecal microbiota profiles in treatment-naive pediatric inflammatory bowel disease - associations with disease phenotype, treatment, and outcome. Clin. Exp. Gastroenterol.12, 37–49 (2019). PubMedPubMed CentralGoogle Scholar
- Backhed, F. et al. Defining a healthy human gut microbiome: current concepts, future directions, and clinical applications. Cell Host Microbe12, 611–622 (2012). PubMedGoogle Scholar
- Dey, M. Toward a personalized approach in prebiotics research. Nutrients9, 92 (2017). PubMed CentralGoogle Scholar
- Healey, G. et al. Habitual dietary fibre intake influences gut microbiota response to an inulin-type fructan prebiotic: a randomised, double-blind, placebo-controlled, cross-over, human intervention study. Br. J. Nutr.119, 176–189 (2018). CASPubMedGoogle Scholar
- Tandon, D. et al. A prospective randomized, double-blind, placebo-controlled, dose-response relationship study to investigate efficacy of fructo-oligosaccharides (FOS) on human gut microflora. Sci. Rep.9, 5473 (2019). PubMedPubMed CentralGoogle Scholar
- Bian, G. et al. The gut microbiota of healthy aged Chinese is similar to that of the healthy young. mSphere2, e00327–17 (2017). PubMedPubMed CentralGoogle Scholar
- Gloor, G. B., Macklaim, J. M., Pawlowsky-Glahn, V. & Egozcue, J. J. Microbiome datasets are compositional: and this is not optional. Front. Microbiol.8, 2224 (2017). PubMedPubMed CentralGoogle Scholar
- Yatsunenko, T. et al. Human gut microbiome viewed across age and geography. Nature486, 222–227 (2012). This study considers the gut microbiome in evaluating human development, nutritional needs, physiological variations and the effects of westernization. CASPubMedPubMed CentralGoogle Scholar
- Marques, T. M. et al. Programming infant gut microbiota: influence of dietary and environmental factors. Curr. Opin. Biotechnol.21, 149–156 (2010). CASPubMedGoogle Scholar
- Claesson, M. J. et al. Gut microbiota composition correlates with diet and health in the elderly. Nature488, 178–184 (2012). CASPubMedGoogle Scholar
- Clarke, S. F. et al. Exercise and associated dietary extremes impact on gut microbial diversity. Gut63, 1913–1920 (2014). CASPubMedGoogle Scholar
- Engen, P. A., Green, S. J., Voigt, R. M., Forsyth, C. B. & Keshavarzian, A. The gastrointestinal microbiome: alcohol effects on the composition of intestinal microbiota. Alcohol Res.37, 223–236 (2015). PubMedPubMed CentralGoogle Scholar
- Jostins, L. et al. Host-microbe interactions have shaped the genetic architecture of inflammatory bowel disease. Nature491, 119–124 (2012). CASPubMedPubMed CentralGoogle Scholar
- Picoraro, J. A. & LeLeiko, N. S. Omes of inflammatory bowel disease: a primer for clinicians. J. Pediatr. Gastroenterol. Nutr.66, 374–377 (2018). PubMedGoogle Scholar
- Weiser, M. et al. Molecular classification of Crohn’s disease reveals two clinically relevant subtypes. Gut67, 36–42 (2018). CASPubMedGoogle Scholar
- Bourreille, A. et al. Saccharomyces boulardii does not prevent relapse of Crohn’s disease. Clin. Gastroenterol. Hepatol.11, 982–987 (2013). PubMedGoogle Scholar
- Van Gossum, A. et al. Multicenter randomized-controlled clinical trial of probiotics (Lactobacillus johnsonii, LA1) on early endoscopic recurrence of Crohn’s disease after ileo-caecal resection. Inflamm. Bowel Dis.13, 135–142 (2007). PubMedGoogle Scholar
- Tursi, A. et al. Treatment of relapsing mild-to-moderate ulcerative colitis with the probiotic VSL#3 as adjunctive to a standard pharmaceutical treatment: a double-blind, randomized, placebo-controlled study. Am. J. Gastroenterol.105, 2218–2227 (2010). PubMedPubMed CentralGoogle Scholar
- O’Toole, P. W., Marchesi, J. R. & Hill, C. Next-generation probiotics: the spectrum from probiotics to live biotherapeutics. Nat. Microbiol.2, 17057 (2017). PubMedGoogle Scholar
- Ridaura, V. K. et al. Gut microbiota from twins discordant for obesity modulate metabolism in mice. Science341, 1241214 (2013). PubMedGoogle Scholar
- van Nood, E. et al. Duodenal infusion of donor feces for recurrent Clostridium difficile. N. Engl. J. Med.368, 407–415 (2013). PubMedGoogle Scholar
- Lee, C. H. et al. Frozen versus fresh fecal microbiota transplantation and clinical resolution of diarrhea in patients with recurrent Clostridium difficile infection: a randomized clinical trial. JAMA315, 142–149 (2016). CASPubMedGoogle Scholar
- Kelly, C. R. et al. Effect of fecal microbiota transplantation on recurrence in multiply recurrent Clostridium difficile infection: a randomized trial. Ann. Intern. Med.165, 609–616 (2016). PubMedPubMed CentralGoogle Scholar
- Halkjaer, S. I. et al. Faecal microbiota transplantation alters gut microbiota in patients with irritable bowel syndrome: results from a randomised, double-blind placebo-controlled study. Gut67, 2107–2115 (2018). CASPubMedGoogle Scholar
- Delaune, V. et al. Fecal microbiota transplantation: a promising strategy in preventing the progression of non-alcoholic steatohepatitis and improving the anti-cancer immune response. Expert Opin. Biol. Ther.18, 1061–1071 (2018). PubMedGoogle Scholar
- Moayyedi, P. et al. Fecal microbiota transplantation induces remission in patients with active ulcerative colitis in a randomized controlled trial. Gastroenterology149, 102–109 (2015). PubMedGoogle Scholar
- Gupta, S., Allen-Vercoe, E. & Petrof, E. O. Fecal microbiota transplantation: in perspective. Therap. Adv. Gastroenterol.9, 229–239 (2016). PubMedPubMed CentralGoogle Scholar
- Reid, G. et al. How do probiotics and prebiotics function at distant sites? Benef. Microbes8, 521–533 (2017). An ISAPP working group article that looks at how microbiome programming in early life influences the gut microbiota communication with distant sites, such as airways, heart and brain, and influences metabolism. CASPubMedGoogle Scholar
- Hiippala, K. et al. The potential of gut commensals in reinforcing intestinal barrier function and alleviating inflammation. Nutrients10, E988 (2018). PubMedGoogle Scholar
- Crusell, M. K. W. et al. Gestational diabetes is associated with change in the gut microbiota composition in third trimester of pregnancy and postpartum. Microbiome6, 89 (2018). PubMedPubMed CentralGoogle Scholar
- Cousin, F. J. et al. The probiotic Propionibacterium freudenreichii as a new adjuvant for TRAIL-based therapy in colorectal cancer. Oncotarget7, 7161–7178 (2016). PubMedPubMed CentralGoogle Scholar
- Zullo, B. A. & Ciafardini, G. Evaluation of physiological properties of yeast strains isolated from olive oil and their in vitro probiotic trait. Food Microbiol.78, 179–187 (2019). CASPubMedGoogle Scholar
- Gonzalez-Rodriguez, I. et al. Catabolism of glucose and lactose in Bifidobacterium animalis subsp. lactis, studied by 13C nuclear magnetic resonance. Appl. Environ. Microbiol.79, 7628–7638 (2013). CASPubMedPubMed CentralGoogle Scholar
- Kostinek, M. et al. Characterisation and biochemical properties of predominant lactic acid bacteria from fermenting cassava for selection as starter cultures. Int. J. Food Microbiol.114, 342–351 (2007). CASPubMedGoogle Scholar
- Hancock, T., Capon, A., Dooris, M. & Patrick, R. One planet regions: planetary health at the local level. Lancet Planet. Health1, e92–e93 (2017). PubMedGoogle Scholar
- Garchitorena, A. et al. Disease ecology, health and the environment: a framework to account for ecological and socio-economic drivers in the control of neglected tropical diseases. Philos. Trans. R. Soc. B.372, 20160128 (2017). Google Scholar
Acknowledgements
Reviewer information
Nature Reviews Gastroenterology & Hepatology thanks H. Szajewska, and the other, anonymous, reviewer(s), for their contribution to the peer review of this work.